I've talked a fair bit about the deployment of nuclear weapons at sea, but very little about the actual effects of nuclear weapons when used against ships. It's time to plug this gap as best I can, although only limited information has made it to the unclassified world, mostly drawing on the Crossroads tests.
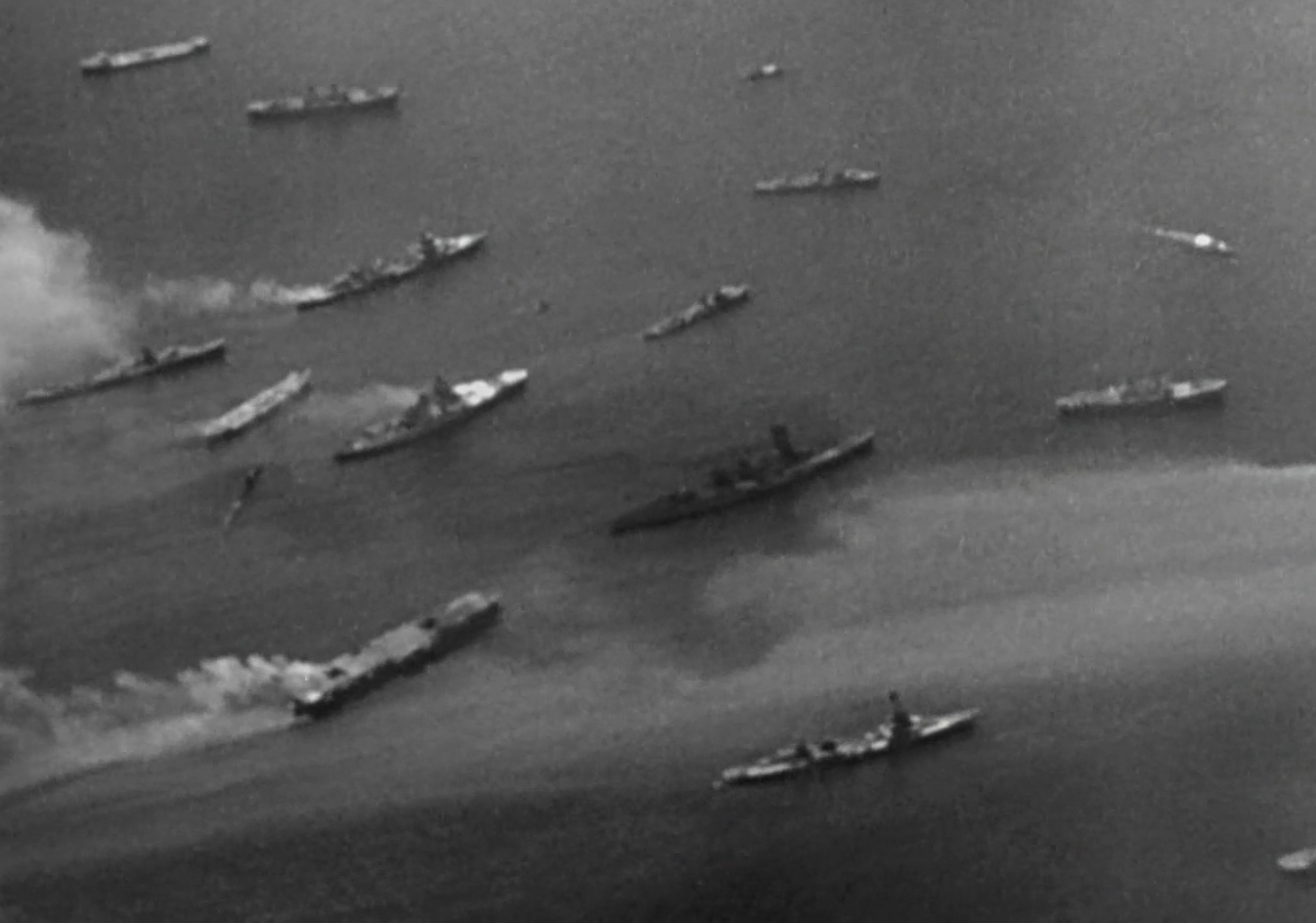
Ships burn after the Crossroads Able shot
Obviously, it's impossible for any ship ever built to survive a direct hit from a nuclear weapon. But as navies evaluated the new weapons, they realized that there were limits to their power. By adopting dispersed formations, and designing their ships to minimize the effects of a near-miss, it would be possible to limit losses to a single ship, or even avoid them altogether if the weapon wasn't placed properly. New communications technologies could make this feasible, allowing ships to coordinate even over the horizon.
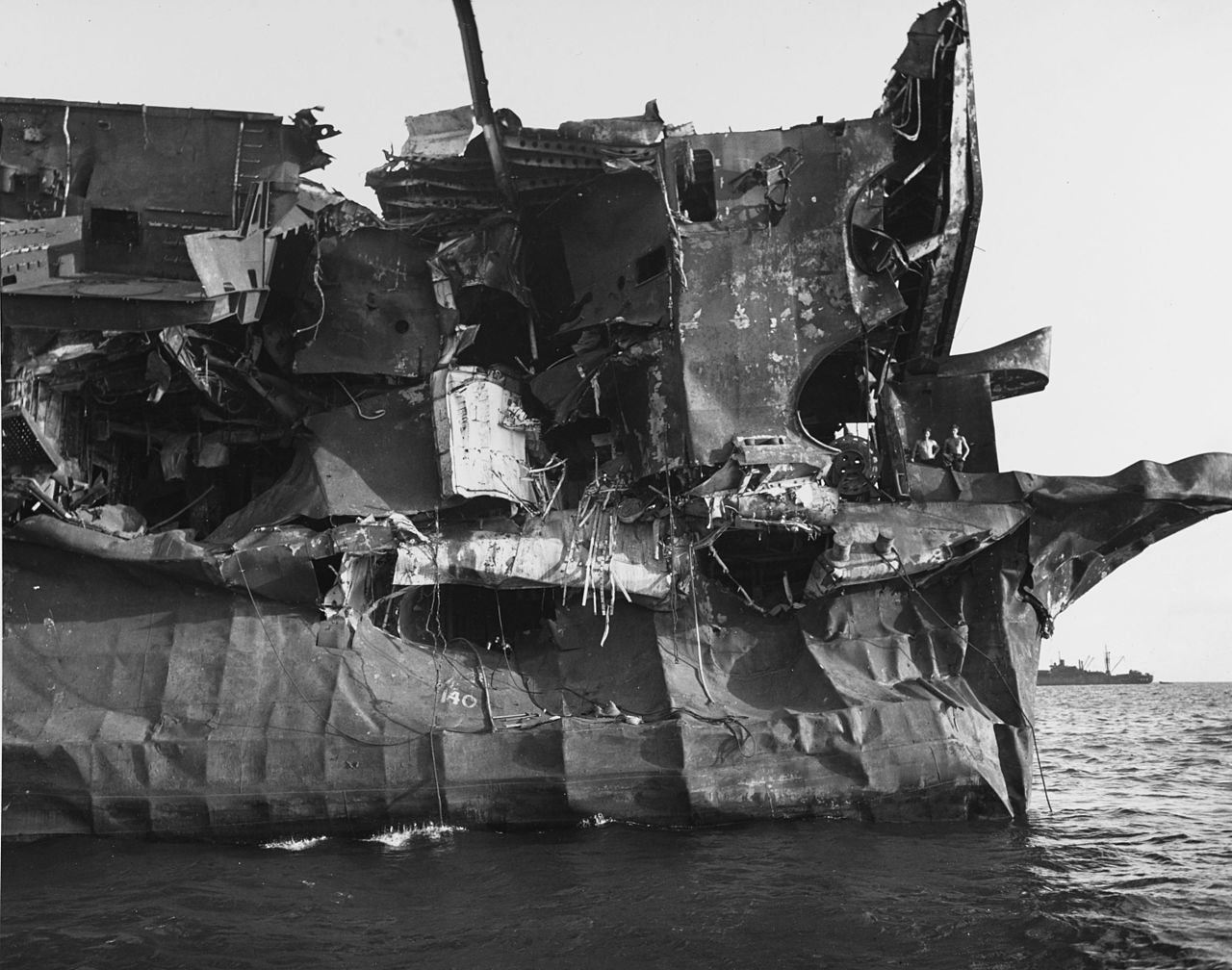
Blast damage aboard the light carrier Independence
Traditionally, the biggest threat to a surface ship has been a nuclear airburst, probably delivered by a missile or bomb. The primary threat from this kind of attack is blast, a fast-moving wall of air that subjects whatever it hits to significant overpressure. About half of an airburst nuclear weapon's energy ends up in the blast, and while the hull of a ship is generally quite resistant to it, the superstructure and antennas aren't. This makes ships hard to sink with airbursts, but much easier to mission-kill. The USN found that a typical hull could stand 50 psi or more (a 350 kT weapon at about .6 miles), hardening the ship's topside to take even 10 psi (350 kT at 1.7 nm) was quite difficult. To prevent the superstructure from being blown off the hull, its sides were extensions of hull bulkheads, accounting for the blocky shape of many postwar US surface ships. Conventional antennas and other topside systems are even harder to protect, although progress was made during the Cold War. Blast can also reach deep into the ship via ventilation paths, either for the crew or for the engines. In the aftermath of the Crossroads test, the USN began fitting specially-shaped funnel caps to reduce this possibility. And even if all of these threats were dealt with, there was still the potential for the blast wave to physically capsize the ship.
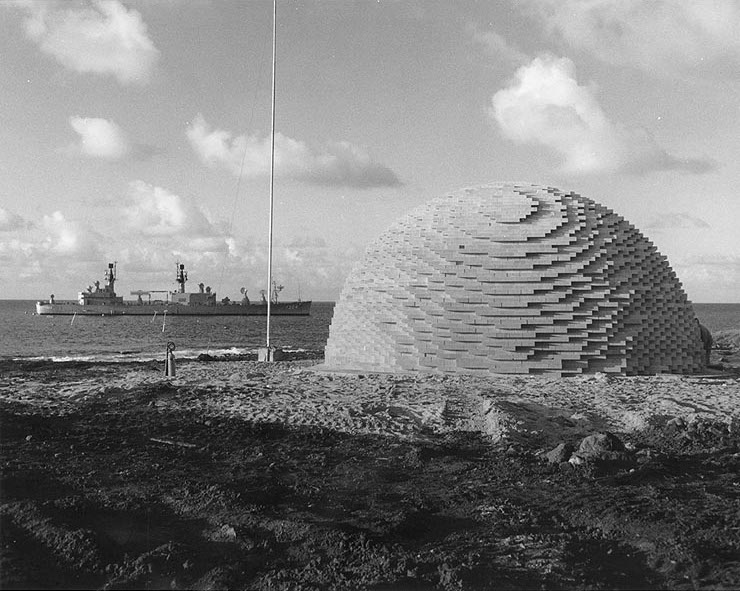
Atlanta with one of the charges for Sailor Hat
Able shot of Operation Crossroads was the only time that ships were directly exposed to airburst nuclear weapons, which meant that when the USN wanted more data in the early 60s, they had to get clever. The WWII-era light cruiser Atlanta was taken out of mothballs and everything above the main deck was removed and replaced with two destroyer superstructures and a variety of radar antennas. Three shots were planned at Kahoʻolawe, Hawaii, each using a 500-ton, 17-foot tall hemisphere of TNT recovered from disassembled weapons. Atlanta would be placed closest to the charge, while modern destroyers were also included, at a greater standoff, to validate their blast-resistance. The first shot of Operation Sailor Hat saw the test ships bombarded by rocks from the shore as well as the blast. Atlanta's crew remained onboard throughout all three tests, although none of them were abovedecks. This was a wise precaution, as the equipment and structures topside was badly beaten up. Fortunately, the tests showed that while detail design improvements were required, there were no fundamental problems with blast-hardening to the desired 10 psi.
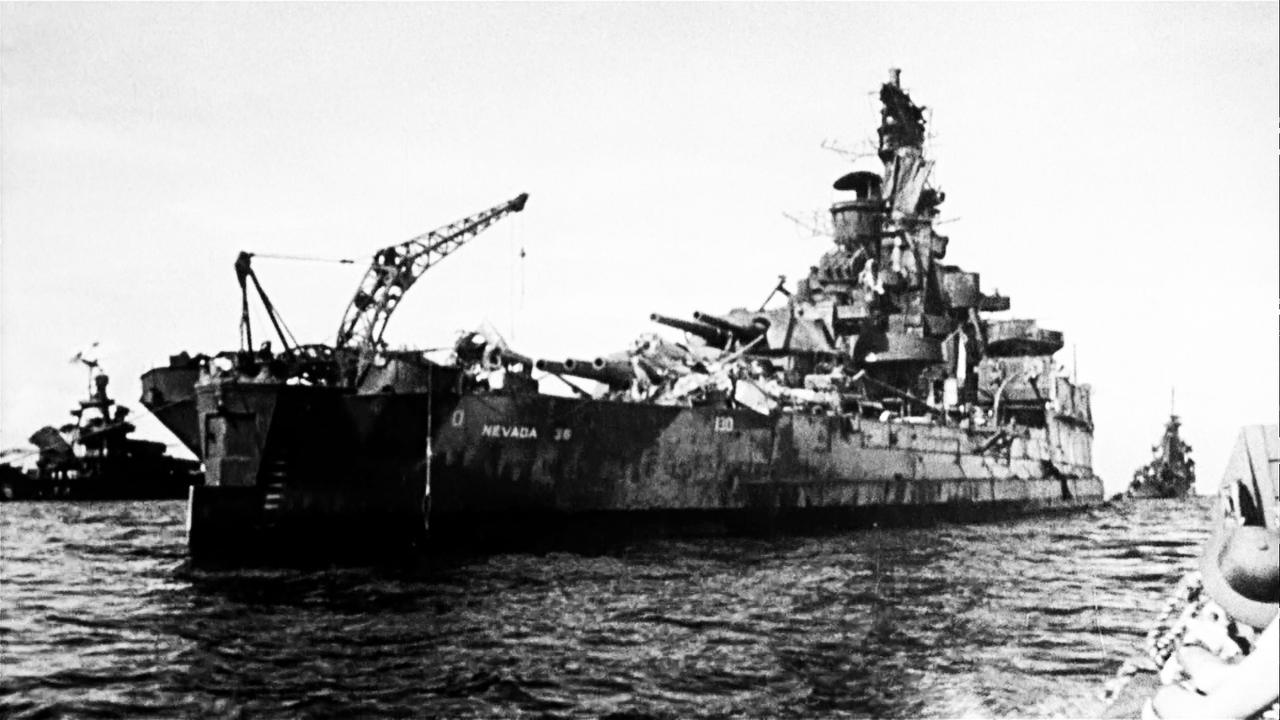
Nevada showing the effects of Crossroads
But blast isn't the only way an airburst can damage ships. About a third of the weapon's energy goes into a massive thermal pulse, and at close range, that might be enough to melt the superstructure, particularly if it is built from aluminum. Our theoretical 350 kT warhead, bursting 1.7 nm away would also deliver 100 cal/cm2, an order of magnitude more energy than is required to cause third-degree burns to exposed skin at that range. Unfortunately, none of my sources indicate what the thresholds are for doing damage to the ship. Radiation is also a concern for the crew, although our standard blast would only deliver about 10 rem to even a fully-exposed sailor, an order of magnitude less than required to cause any symptoms of radiation sickness. Note that, for a given blast pressure, a larger, more distant weapon will generally expose the ship to more thermal energy, but less radiation, while the inverse is true for a smaller weapon.1
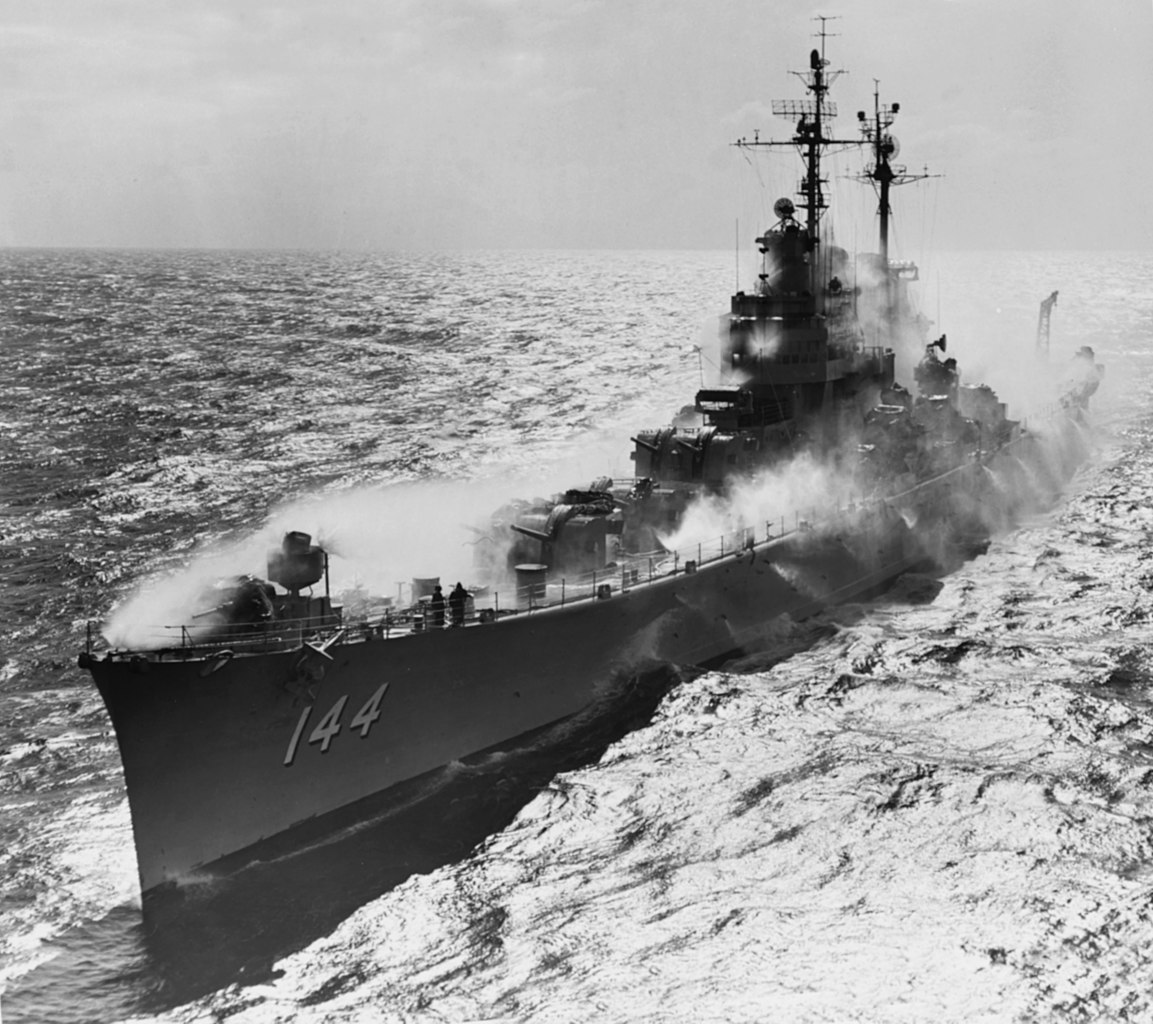
USS Worcester shows her washdown system
A bigger problem than direct radiation is fallout, the radioactive debris left by the weapon. Underwater bursts produce particularly vicious fallout because the debris from the weapon gets deposited locally instead of dispersed in the atmosphere. The contamination from the Crossroads Baker test was enough to send naval architects to work on countermeasures. The standard method was a washdown system, a set of sprinklers built into ship that would be activated before the attack. In combination with careful design to make sure that fallout didn't get trapped somewhere, such as sharp deck edges to get water cleanly off the ship, this would hopefully allow the ship to be fought after passing through a fallout cloud. Obviously, crew couldn't stay in the open during this process, and things like open bridges were largely abandoned. Some navies took this even further, building a "citadel" that is provided with full filtration capabilities, and making sure that the ship can be run entirely from within it. This in turn drove the adoption of automated guns, and provided protection against not only fallout, but also chemical and biological warfare.2
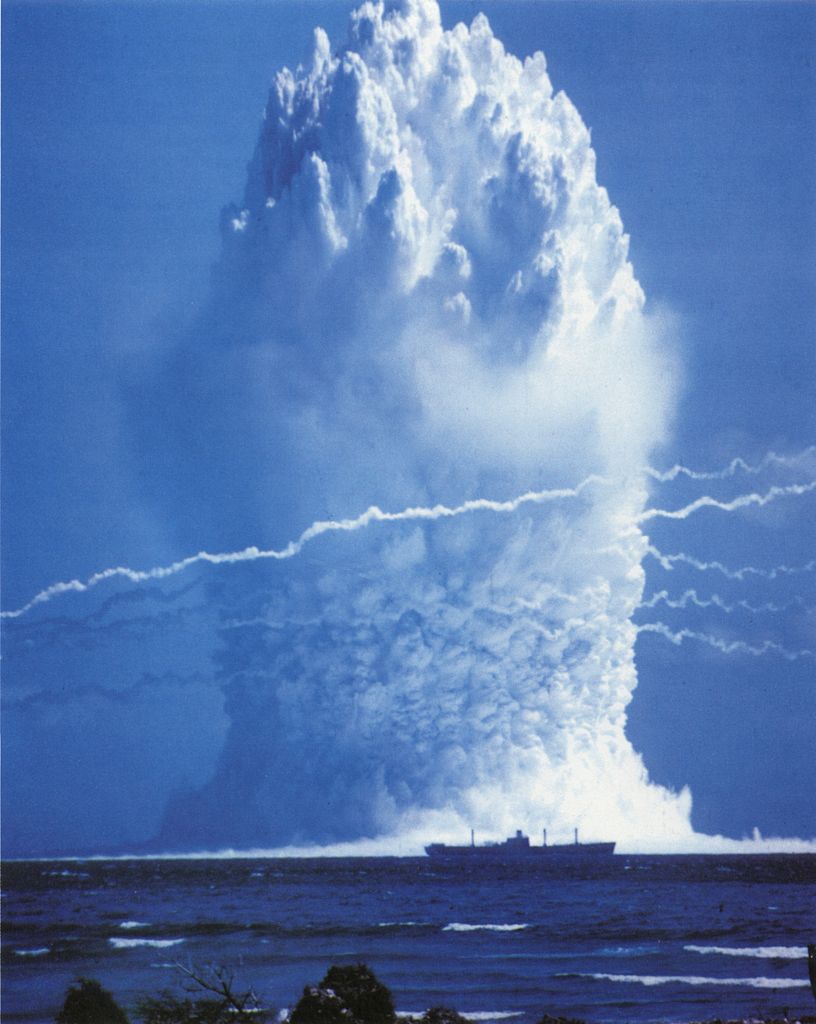
Hardtack Umbrella throws a fountain of water skyward
Underwater nuclear detonations have other effects besides contamination, of course. The most important is a massive shockwave, which, thanks to the greater density and reduced compressibility of water, produces much greater overpressures than an airburst does, although for a shorter time period. This meant that a nuclear depth bomb (NDB) could be lethal against submarines within a surprisingly large radius, although this radius shrank over time as submarines became able to dive deeper. Even a relatively small NDB, like the 10 kT B57, would probably be able to destroy or disable a typical 50s submarine within a mile of the detonation, although a 60s-era SSN is likely to require the bomb to fall within a thousand yards or so for an assured kill.3
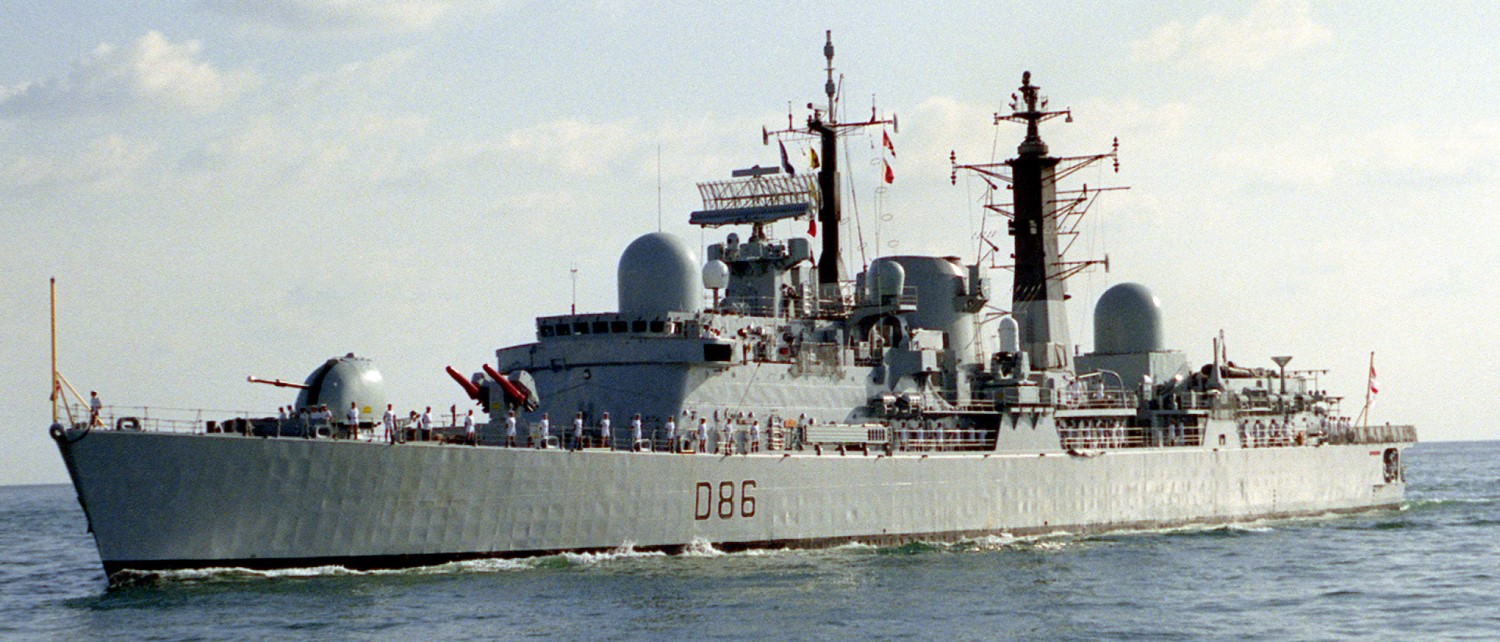
HMS Birmingham displays "hungry horse", a dimpling of plating between the frames resulting from building for nuclear shocks
But submarines aren't the only things that might be impacted by underwater nuclear bursts in the neighborhood. These were most likely to be side-effects of ASW efforts, although the Soviets did use a few nuclear anti-ship torpedoes. The problem was that submarines by nature are heavily hardened against pressure, while surface ships are not. The overpressure from the 10 kT W44 of ASROC would be just shy of 100 psi at the maximum range of the weapon, a potentially serious problem for the launching ship.4 While underwater explosions had long been a problem, nuclear shocks were unique in hitting the whole ship at once instead of being localized. The result was a much greater focus on shock resistance than had previously been common. Hull construction moved towards more frames and thinner skin, which allowed the ship to flex with the explosion. Supports for heavy items like boilers and turbines were beefed up, and lighter objects secured and tested to see how they handled shock. Even aircraft might not be immune if the depth charge went off just below the surface, as some of the blast wave would escape into the air, followed by hundreds of thousands of tons of water. The Soviets estimated that a helicopter delivering an NDB had only a 50-50 chance of survival.
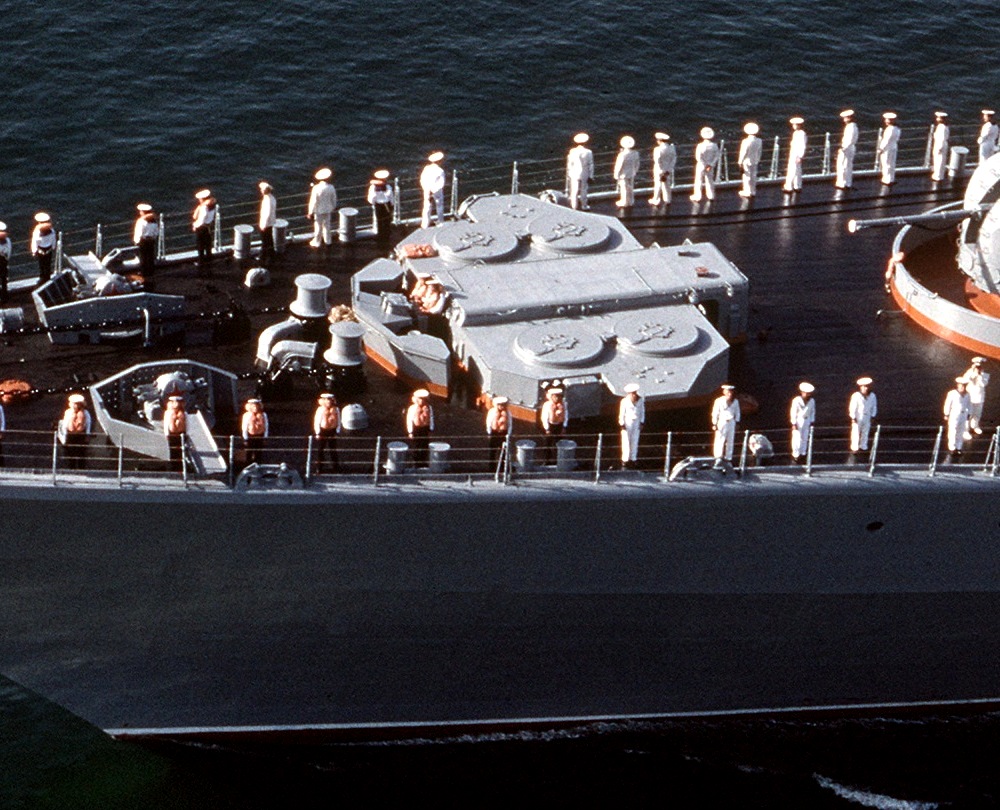
Soviet sailors with the glass-fiber lifelines of a Udaloy class destroyer
One last effect that bears mention is the electro-magnetic pulse (EMP) generated by a distant nuclear explosion. This is a strange and unpredictable effect, and while stories of tests in the distant Pacific knocking out power in Hawaii are true, they usually fail to mention that it only knocked out a few areas, leaving most of the island unharmed. In practice, most military electronics have at least some hardening, although designers do make efforts to remove any potential antennas that EMP might latch on to. The Soviets took special precautions, replacing the metal lifelines at the sides of the ship with glass-fiber versions, which not only removed a potential source of EMP damage, but also reduced radar signature and improved radio range.
Fortunately, nuclear weapons were never used in anger at sea, and most that might have been are long retired. But the possibility still exists, and modern warships, while they may not be expected to use nuclear weapons, are still designed to resist them.
1 I'm not actually sure if this means that the thermal radiation is more of a problem for the bigger weapon. Bigger bombs deliver their thermal radiation over a longer period of time, and so require higher cal/cm2 values to achieve a given effect. ⇑
2 The US was a late adopter of this technology, with it first entering service on the Arleigh Burke class. ⇑
3 Information comes from here, which goes into more detail on both conventional and nuclear depth charges. ⇑
4 It's possible that I'm overestimating the shock wave, as it appears that it decreases after it reaches the surface, and a surface ship by definition sits on the surface. It's worth pointing out that the destroyer Agerholm fired an ASROC and appears to have survived without problems. ⇑
Comments
I doubt the time the thermal radiation is applied for is going to have much all that much of an effect given how long it'll take for the hull to come to thermal equilibrium.
Also metals lose strength when heated so even without melting it might reach the point at which it just isn't strong enough to withstand the blast wave which will come after the heat even if it would've easily withstood it cold.
The hull doesn't have to come to thermal equilibrium for the duration of the thermal pulse to make a difference. I'd guess that you can get significant radiation during the length of the pulse. The effect is definitely real. For instance, a 35 kt weapon requires about 32 cal/cm2 to ignite paper, a 1.4 Mt weapon takes 48 cal/cm2, and a 20 Mt weapon 85 cal/cm2. I just don't know exactly how it works on warship hulls.
Did they ever consider painting ships in anti-flash white?
Correct me if I'm wrong: 100 cal cm-2 ≡ 4.18 MJm-2
Steel has a specific heat capacity of roughly 0.5Jg-1K-1 and a density of 8Mgm-3 Therefore 1m3 of steel has a heat capacity of around 4MJK-1
Thus the above thermal flux is capable of heating steel sheet by 1.04Km If we assume steel loses most of its strength by 600°C, the flux will be enough to defeat a maximum of 1.8mm of steel. It will be able to melt at most 0.78mm of steel.
I hope the markdown works.
I don't know of any plans to paint ships anti-flash white. I doubt it was seriously considered, because nuclear warfighting was never the only purpose of warships, unlike SAC's bombers, and white doesn't work that well for visual camouflage.
As for steel, thanks for the numbers. Worth pointing out that typical warship steel is probably at least 10 lb/.25", or something a bit over 6 mm. 100 cal cm2 doesn't seem likely to do too much to even that, although if it's marginal on blast, the weakening might have an effect.
As an addendum to Lambert's math, aluminum has roughly twice the heat capacity of iron, and roughly twice the heat of fusion. However, its melting point is less than half as far above room temperature. Doing the math, it takes slightly more energy to melt aluminum than iron, 985 J/g versus 947 J/g. However, it's also about a third as dense, so the same thermal energy can defeat a little less than three times the thickness of aluminum. Call it 2mm or so melted.
Melting damage doesn't seem to be a big threat to a ship's survival, unless the bomb is so close that it's already destroying the ship in some other fashion. However, that kind of thermal pulse could easily play merry hell with anything sensitive that's exposed to it, like electronics.
The risk with having heat applied to the surface of a steel plate at a very high rate of MJ/sec, is that even if the total amount of heat is insufficient to heat the entire plate to a dangerous degree, you might heat the surface 0.5 mm or so by several hundred degrees for a fraction of a second before that heat is conducted away into the depth of the steel.
This is deliberately done in some industrial processes, because it gives a very rapid rise then fall in the temperature of that thin layer.
The result is much as if you heated an entire piece of steel to that temperature, and then plunged it into water to give a rapid quench. Not to mention that on a ship, actual plunging into water is also on the cards. (To rub salt in the wound, sea water produces a faster quench than fresh.)
A rapid quence of hot steel can (depending on the exact temperature/time curve and the previous state and composition of the steel) give you something that is very brittle and easy to crack.
Now this is just the very surface layer, but we now have a phenomena called surface embrittlement, where a thin surface layer of brittle material can generate a high speed crack, which can then grow deep into the tougher subsurface material, causing the entire part to fail. A good way to induce this would be to hit the brittle surface with a very sharp impact. Such as a nuclear blast wave.
To some extent, this could be addressed by careful changes in the steel alloy composition. But if this was done I would suspect that it's probably not public information.
@Alsadius,
Don't get distracted by melting point, what matters is the temperature at which the strength starts to plummet. (This is the error that 911 "truthers" made. Well, one of many errors.)
See here: https://firesciencereviews.springeropen.com/articles/10.1186/s40038-015-0007-5 especially Fig. 9.
You can see that by 250 degrees C the strength of a typical Aluminium alloy is half that of room temperature, and falling rapidly. Not what you want when a blast wave is seconds away.
Also, is it just me, or does the Sailor Hat charge look like something from Minecraft?
@Doctorpat: Yes, I know that melting alone is not the likely failure mode here. (That was the conclusion of my comment, after all.) It's merely an easy number to calculate, and I'd say it gives a fairly intuitive sense of what the simple, direct effects of the heat will be. And I think the conclusion I reached - that if they're a threat, the ship is already dead - is basically in line with your claims, broadly speaking.
Indirect effects, like those you outline, are a whole different ball game. I'm not enough of an expert to speak on those(and it seems you may be, so I won't add anything further for fear of making a fool of myself).
Curious – is this true even with neutron bombs?
(Does saying "enhanced radiation weapons" instead make me sound more informed/less like an uninformed nut raving about how evil it is to make a Magic Death Ray Bomb, or just make me sound more like someone whose job is to create jargon to turn into acronyms?)
Weirdly, at least a little bit. To an extremely rough approximation, an enhanced radiation weapon/neutron bomb (both are reasonably correct) is a standard weapon with about 6x the radiation and otherwise similar effects of a bomb that's about 75% of it's rated yield. So a 480 kT neutron bomb would have the effects discussed above, and would deliver 60 rem, still too little to actually cause radiation sickness. But this is all very approximate, because many of the effects involved are nonlinear. Neutrons are absorbed by the atmosphere, and given that hydrogen is a great neutron absorber, I wouldn't be surprised if the humid air near sea level made ERWs fade off even more quickly than usual. The theory behind them is that tanks are very hard to kill with nuclear weapons, and biasing the weapons towards neutron radiation would make them more effective at killing tanks. But ships are much less hard, and there are better ways to kill them.
It strikes me that if thermal effects were important, cold war ships would have been coated with some sort of ablative material.
Didn't the farm animals at Crossroads show that prompt radiation was a threat to sailors?
Also I hear that aside from the overstated effects of EMPs, radar equipment tends to work better when there's not a big ball of conductive plasma in the sky. Are ships temporarily blinded and does this last long enough for the attacker to take advantage?
The problem is that the ablator is going to be a major source of topweight, and interfere with the usual routine of painting the ship. I believe the washdown system would provide some protection.
Not really. Most of the animals survived the prompt radiation, and died due to starvation when the ships couldn't be boarded again.
Re radar effects, I don't think this is as big a deal as it's made out to be. The conductive plasma doesn't last that long unless it's in the ionosphere, and if it is, then it really only matters for signals that meaningfully interact with the ionosphere. I strongly suspect that modern signal processing makes that a nonissue in most cases, and I've heard that there were good solutions back in the 60s.
The duration of the thermal pulse from a nuke is something like 0.4-20s, with larger yields giving longer pulses. Using Lambert's nominal flux of 100 cal/cm2 = 4 MJ/m2, this corresponds to an intensity of 0.2-10 MW/m2.
Lambert found this flux would be sufficient to heat a ~1mm steel panel to its melting point (1800K; 1500K above ambient). The thermal conductivity of iron is 80 W/(m.K), so this heat flux would be conducted through the panel on a timescale of (0.0014e6) / (150080) = 0.03 s.
This is shorter than the duration of the thermal pulse, so with these figures, we're not in the regime in which the surface of the ship (including e.g. fasteners) would melt and flow before the heat can be distributed deeper into the structure. But it's within 1-2 orders of magnitude, so Doctorpat's scenario - surface heating of a few hundred K, and resulting embrittlement - is realistic.
@DampOctopus
That's a much slower pulse than I was expecting, but at the short end sort of like the numbers you'd see in industry for surface hardening processes.
eg. Laser hardening (the one most similar to nuclear flash hardening (TM) ) is done over a total irradiation time of eg. 0.2 seconds https://www.researchgate.net/publication/264815656LasersurfacehardeningA_review#:
The length of the pulse surprised me, too.
If you look at the plot in the link I provided, you'll see that there are actually two pulses: the first, fast pulse is produced from a volume of air heated by x-rays from the initial, compact, high-temperature fireball; the second, slower pulse comes directly from the fireball, after it's expanded and had time to cool so it's emitting primarily in visible/infrared. The second pulse continues until the fireball has radiated away most of its energy - which can take a while, for a big blob of optically-thick material. (Caveat: I'm not an expert, and may have misunderstood this.)
I've ignored the first pulse, because it contains much less energy and has only slightly higher peak power ... at least, in the example in the plot. In practice, this will probably be yield- and altitude-dependent, though I'm having trouble figuring out which way those factors will affect it. So there might be some circumstances under which the initial fast (~10s of milliseconds) pulse is more important in terms of weapon effect.
Incidentally, if you wanted armor that was effective against a nuclear thermal pulse - or any sort of pulsed directed-energy weapon - I think this is an argument for making it semitransparent, so the deposited energy is spread throughout the depth of your armor rather than being concentrated at the surface.
If it's semi-transparent that it's going to affect whatever the armor is meant to be protecting.
Probably better to go for something that intrinsically has low absorption in whatever wavelength the heat you're expecting will be, assuming you can find such a material that meets the other requirements.
I'm assuming the length of the pulse is for the same reason that red giants are brighter than main sequence stars despite being cooler: the lower emittance is counteracted by the larger area.
Also the whole 'air going opaque at a few kK' thing.
https://www.rand.org/content/dam/rand/pubs/papers/2006/P3026.pdf This is the most comprehensive paper I can find on why the thermal radiation curve is like that.
Thanks - that paper's really clear and informative. It also shows that all this physics was well understood back in the 1960s.